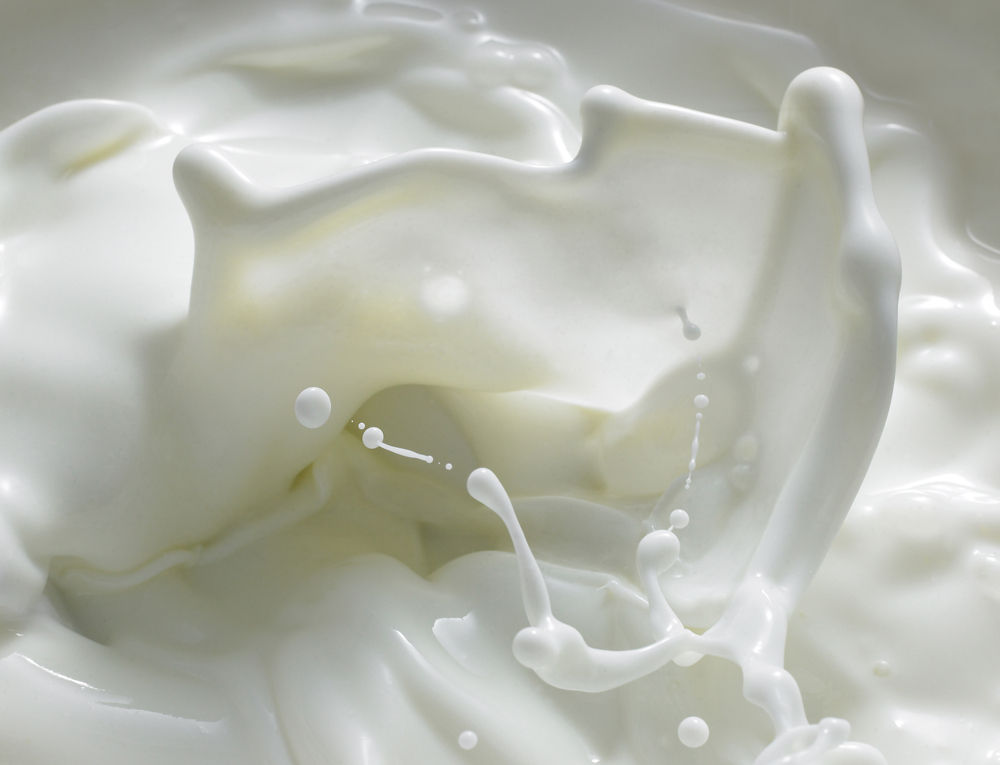

Physicochemical
TECHNOLOGICAL & FUNCTIONAL PROPERTIES OF FOOD PROTEINS
Dairy proteins represent about 30% of the total dietary proteins of adults in Western countries. Although these proteins in their native state have as one of their functions the transport of molecules, they are present in food in several structural states, reflecting the variety of processing used industrially.
Although the impact of the structural alteration of milk proteins on the techno-functional properties of foods (viscosity, etc.) is known, the understanding of their effect on the functional properties (health claims) of these foods is still under construction. It is a consensus that food matrices are not inert to the addition of bioactive compounds, yet this fact is ignored during the development of functional foods, generally based on the simple incorporation of these compounds in foods. In order to guide the development of functional foods and guarantee the associated beneficial effects, it is crucial to understand how structural changes induced to protein food matrices affect the biological activity of bioactive compounds. In this context, the present research project proposes a reverse engineering strategy to generate knowledge that allows to predict the behavior of carotenoids in protein matrices submitted to different industrial processes. Through the association of results obtained in real matrices and model, the aim is to establish generic rules for the behavior (interaction / biological activity) of carotenoids in complex multi-protein matrices. In addition to the scientific community, the knowledge generated by this proposal will also interest companies in the area of functional foods / ingredients, as well as regulatory bodies.
MICROSTRUCTURE OF DAIRY PRODUCTS
Food technology can be defined as a controlled attempt to preserve, transform, create or destroy a structure that has been transmitted by nature or by processing. There are currently numerous characterization tools available to probe the structure of food in order to rationally design processes that improve the quality of products.
A characteristic of interest for the solution of problems and flaws currently in evidence, is in the identification and characterization of the micro and nanostructures of milk and dairy products. Figure 1A summarizes the main components of milk organized by the average size of its hydrodynamic rays, while figure 1B shows the main structures of milk and also of the ingredients and particles most commonly used and found in the technology of processing dairy products.

Figure 1: characterization of the micro and nanostructures of milk and dairy products: (A) average hydrodynamic diameters of the main structures present in milk in micrometers; (B) main particles found in dairy products
The occluded air in the atomized particles directly affects the final quality of powdered milk, such as, for example, low volumetric density and formation of fines. The incorporation of air in the concentrate occurs at several points, and the greater the foaming ability, the greater the ability to incorporate atmospheric air.
During drying, there is an increase in the concentration of solids, viscosity and surface tension of the particles, forming a layer on the outside that makes diffusion difficult. This becomes more pronounced as drying progresses and when the humidity of the particles reaches around 10% to 20%, which is the so-called "critical humidity point", the temperature of the particles gradually increases with drying. However, if the temperature of the air surrounding the particles is high enough, overheating may occur, denaturing the protein, which influences the inadequate solubilization of powdered milk and the hardening of amorphous lactose which prevents the diffusion of steam. Thus, with steam and air remaining inside, the particles expand by pressing on the outer layer, swelling like a hollow sphere with a layer thickness around 1 micrometer, in some cases, explosions, as shown in figure 2.

Figure 2: Morphological data of whole milk powder, with the presence of particles with characteristics of explosions.
Another extremely useful application of the techniques concerns the characterization of dehydrated products. On the surface of the whole powdered milk particles are small fat globules that are not protected by the lipoprotein membrane, called free fat, which can cause problems, such as product rancification when stored for a long time, agglomeration of particles and difficulty in reconstitution.
As shown in figure 3, the inefficiency of the homogenization process of concentrated milk aiming at the production of whole milk powder, had a direct influence on the amount of free fat, which tends to involve air cells in the powdered milk particles or form “eruptions” ”On their surface, promoting oxidation and even agglomeration of the powder
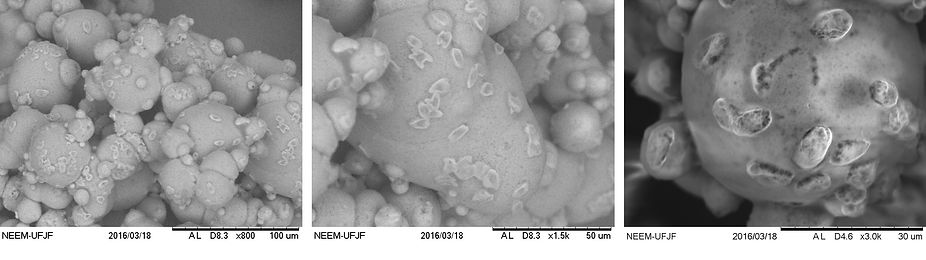
Figure 3: Morphological data of the surface of whole powdered milk particles, with the presence of free fat migration, caused by inefficiency of the homogenization process of concentrated milk, generating agglomeration in the product. .
The shelf life of dehydrated dairy products is generally established to ensure microbiological safety and to maintain sensory characteristics acceptable. Despite being microbiologically stable, physical and chemical changes, such as lactose crystallization, particle agglutination, fat oxidation, Maillard reaction and enzymatic reactions can occur during storage, changing the product's physical-chemical and functional properties.
In the last few decades, spectroscopic methods, together with chemometric tools, have been applied to evaluate the quality of dairy products as an alternative to traditional procedures. Figure 4A shows the Raman spectrum of the WPC produced under ideal conditions, 4B WPC produced under ideal conditions and stored for 6 weeks under ambient conditions and 4C α-lactose monohydrate.
The changes that occur in the Raman spectra of samples stored in ambient conditions are caused, mainly, by the increase in the amount of water in the powder. When the WPC is exposed to high relative humidity, the powder absorbs water and there is an increase in its concentration in the food. As a consequence, there is a decrease in viscosity and an increase in molecular mobility. These two factors determine a series of time-dependent structural modifications, such as: guminess, collapse and crystallization of amorphous components, which shorten the life of the WPC and, consequently, its quality.

Figure 4: Raman spectra of WPC produced under ideal conditions (A), WPC produced under ideal conditions and stored for 6 weeks in ambient condition (B); α-lactose monohydrate (C).
Another technique for investigating the microstructure of powder products, used during the rehydration process is the analysis of particle size by laser diffraction, which is widely used in several industrial sectors due to its ease of operation, speed and amplitude of reading.
Figure 5 shows, for each sample of whole milk powder with different characteristics of agglomeration and manufacturing process, the percentage distribution of the volume occupied by the particles (during the rehydration process in water) according to their hydrodynamic diameters. Typically, the particle size distribution of whole fluid milk shows two populations, one corresponding to the casein micelles (centered around 150-200 nm) and another corresponding to the fat globules (centered around 5 µm). All samples have a population of particles centered at approximately 200 nm, which correspond to the casein micelles as in fluid milk. The intensity of this population depends directly on the quality of the powder's microstructure.

Figure 5: Size distribution of powdered milk particles with different microstructure characteristics during the rehydration process: A (□), B (○), C (Δ), D (◊) and E (●).